Physical mechanisms of development and evolution: An interview with Stuart Newman
by Stuart A Newman & Katrina J Falkenberg
18 September 2018
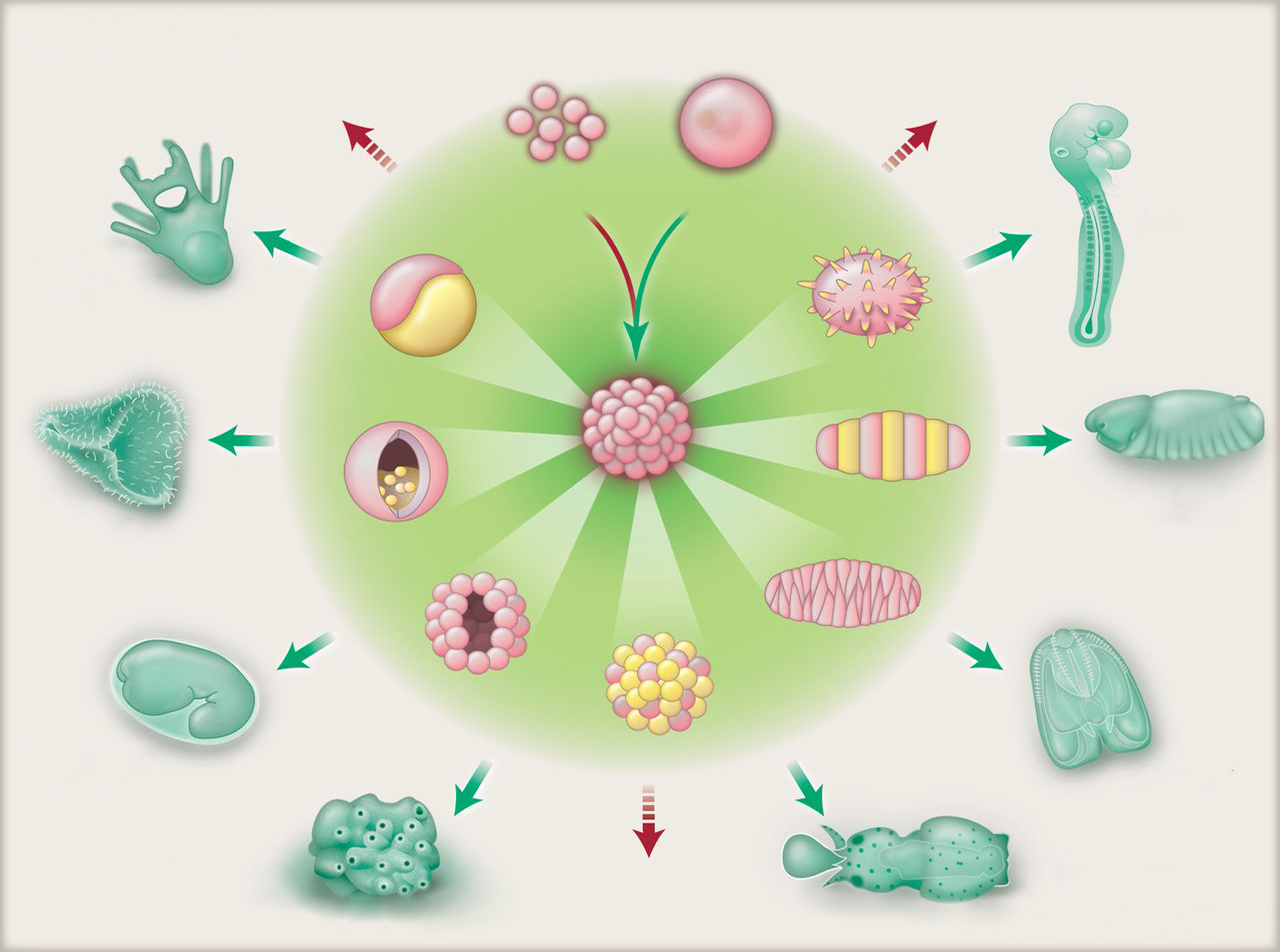
Stuart Newman is a Professor of Cell Biology and Anatomy at the New York Medical College in Valhalla, New York. He is a prominent evolutionary developmental biologist, well known for his pioneering work on vertebrate limb development and the role of physical mechanisms in development and morphological evolution. Katrina Falkenberg is the science communication and outreach officer for the EES research program. She interviewed Stuart about physical mechanisms of development and their importance for understanding evolution.
Katrina: What sparked your interest in the generic physical mechanisms of development and evolution? Are there certain people who were particularly influential for you?
Stuart: Let’s start by defining generic physical mechanisms. These are morphogenetic and developmental patterning processes that produce similar outcomes (because they are causally similar), to physical processes affecting the behavior of nonliving materials. When I started out in developmental biology, there was a very prevalent idea that organisms develop by genetic programs. It was thought that if you could somehow understand enough about the interactions among genes and their products, you would have a program for how the organism develops. In the 1970’s there was a tremendous burst of activity in this area. There was an idea that the evolutionary history of an organism provided it with a little representation inside every cell about where every part should appear. Some people considered the cybernetic idea of positional information, whereby molecular signals could elicit parts of the stored representation according to an evolved program. On this view, there are no common principles of how to build an organism. There’s nothing less generic than that because every organism is different. Development was viewed as a program that was arrived at by evolution.
There were other ideas floating around at the time. For example, D’Arcy Thompson’s book On Growth and Form (see a previous blog post) came my way during the 1970’s. Back in 1918, Thompson was suggesting that the forms of organisms were a result of the physics of the materials that made them. Unfortunately D’Arcy Thompson’s ideas were put aside and marginalized because of the intervening genetic revolution. Another influential figure was Alan Turing. In the early 1950’s, Turing proposed the idea of reaction diffusion systems. He wrote a famous paper called the Chemical Basis of Morphogenesis where he talked about this physical mechanism of diffusion and chemical reaction that under certain circumstances can produce a non-uniform distribution of a molecule. This means you can get morphogenesis out of simple physical interactions. Even though this was theoretical biology – it hadn’t even been shown in chemical systems at that point – the idea still hung around. There was also the work of Malcolm Steinberg who studied adhesion in embryonic tissues. He found that tissues could behave like liquids and therefore undergo phase separation (like oil droplets in water), and tissue layers could form because of their liquid properties. Steinberg also had trouble getting his ideas into the mainstream because people were captivated by the new genetics.
The Darwinian paradigm is that everything complex has to take an extremely long time, but in fact, Stephen Jay Gould and his co-workers were writing about sudden and abrupt appearance of forms, and later, about the Cambrian explosion. It seemed like some determinants were there very early in evolution that produced forms that had similar properties across many different phyla. I came out of theoretical chemistry and theoretical biology, and looking at all these models, I could see some features of organismal development that were indeed generic, such as these liquid-like properties and reaction diffusion properties. I started to think about how we could go, not away from the gene, but beyond the gene. What other determinants could give rise to forms?
Katrina: Where did your work on physical mechanisms of development start?
Stuart: With a background in physics of materials, I started thinking about developmental problems in terms of physics. One of the first problems I addressed was the vertebrate limb. There is a harmonic periodicity to the limb, with an increasing number of elements as you move from the body outwards towards the fingers. And there’s a wave-like repetitive pattern to these structures that look like copies of each other. At the time I was working on this, the positional information group was particularly interested in why the fingers were different from each other (e.g. why is finger number two different from finger number three, etc.), but the thing that compelled me was why are they the same as each other. The differences seemed to be fine tuning.
My theoretical physicist colleague, Harry Frisch, and I produced a model for the vertebrate limb which we published in 1979, that explained the pattern of the limb as a harmonic series of standing waves. We drew on Turing’s reaction diffusion mechanism to do that. It created a little bit of interest but I think the alternative positional information group was so dominant at the time that this work was on the margins for many years. It was revisited 35 years later by other groups using new methodologies and now it seems to be the mainstream idea about how the limb develops (recent review).
I also started working on physical interpretations of the Cambrian explosion, the burst of morphological disparity early in evolution. It seemed like there wasn’t enough time for very gradual genetic mechanisms to develop all these forms and the idea appealed to me that their genes weren’t all that different from each other. At the time, our knowledge of the developmental genetic toolkit – the common genes that are active in morphogenesis across the animal kingdom – was deepening and we were becoming aware that genes are in fact very conserved. I thought that genes might mobilize physical forces in different ways in different lineages, so with essentially the same set of genes, you can generate a multiplicity of forms. Therefore, the generation of disparate forms wouldn’t take that much time because all the genetic information was already there, it’s just used differently. I don’t simply mean a long series of minor modifications in sequence that may fine tune gene regulatory networks – that is, a purely gene centered approach – but that the use of the genes to create forms employs physics. Although there’s a sense that physics comes for free and it’s part of the natural world, not every material is susceptible to the same physical processes. Liquids have a different physics than solids. Gases have a different physics than either of those two. And the question arose, what does it take for a material, a living material, a cluster of cells for example, to behave in a certain physical way? The subsequent work that my colleagues and I did in this area explored those questions.
Katrina: Is it a correct interpretation of your work to say that generic physical mechanisms came early in evolutionary history, they produced some viable forms that were selected for, and what we see today is genetic accommodation of those early forms?
Stuart: I think it’s basically right. But I don’t want to encourage the misinterpretation that physical determinants operated independently of genes in the origination of form because in my work, I start with cells. And the cells have had several billion years of genetic evolution behind them. The interpretation that I’m presenting of the origin of complex forms doesn’t address the origin of cellular life. My works deals with the multicellular holozoans that we call animals. Certain genes mobilized physical processes to produce forms, and those forms emerged very early, and they have been refined over time. Not that many new forms have been innovated subsequently. The generic properties were very determinative early on, and later, genetic evolution consolidated and refined the early-appearing forms.
Let’s take cell adhesion as an example. Having the appropriate adhesive proteins is the primary determinant of going from a unicellular form to a multicellular form. Let’s say they’re calcium dependent for example, but the calcium in the environment is low. Then all of a sudden, the calcium in the environment rises for some geological reason and you have cells sticking together. That’s a major morphological transition and it’s based on physics. But of course it’s also based on pre-existing genes – you can’t do it if you don’t have adhesive proteins. In all metazoan (animal) lineages, the most important adhesive proteins early in evolutionary history are the classical cadherins. These are calcium-dependent adhesive proteins that span the cell membrane, binding neighboring cells to one another while linking each cell to the motile machinery inside it. Until you had classical cadherins, you didn’t have animals. Single celled organisms that preceded the animals had protocadherins, but these didn’t link to the cytoskeleton and therefore couldn’t create the kind of cohesive materials that are the basis of animal embryogenesis. Interestingly in plants, there is a much bigger variety of different adhesive proteins that bind cells together and produce multicellular plants of different lineages (multicellular algae, wild land plants, etc.). It’s true that if you have a physical effect like adhesion, anything that’s sticky could do it, and in fact, many things that are sticky do it in plants. But the earliest and most important ones in the metazoan lineage are the classical cadherins.
Another example is the repurposing of a molecule secreted by a unicellular organism to attract prey. In a clump of cells, the factor is no longer necessarily just acting outside the organism, it’s acting inside the organism. It can become a developmental gradient; a morphogen. You have the recruitment of pre-existing gene products to do certain physical functions, and you can get forms and patterns that arise in this way.
Katrina: Could you walk us through an example of animal evolution that can be explained by these physical forces acting early in evolutionary history?
Stuart: One enigmatic problem that we addressed with these ideas was the process of body segmentation in the long germ band insects, such as flies and mosquitoes, versus the short germ band insects, such as beetles. In long germ band insects, the nuclei are in a syncytium (a common cytoplasm) and seven stripes appear which become a kind of a template for later subdivision of the embryo. A lot of important work done on the genetics of that process led to a Nobel Prize for Christiane Nüsslein-Volhard and Eric Wieschaus.
At the same time, people were looking at short germ band insects, and they seemed to generate their segments in a completely different way. There was a kind of a budding off from one end as the embryo grew, so instead of simultaneous segmentation, there was progressive segmentation. These insects are not that different from each other genetically and evolutionarily, and it became pretty well established that the short germ band predated the long germ band. So how do you go from one to the other?
I suggested that if you look at oscillatory processes, like chemical oscillations, you can build a segmented structure by growing and oscillating at the same time. If you look at the relationship between oscillations and the Turing standing wave mechanism, they are very close to each other mathematically. With very little evidence, I suggested that the short germ band insect is based on an oscillation and growth. And then the long germ band insect was what happens when you put this oscillation in the syncytium so that you get diffusion of the transcription factors along with feedback reactions. This was in the early nineties, even before the oscillatory mechanism of vertebrate segmentation had been elucidated, so it was a complete speculation.
Aspects of this interpretation have proved true. Short germ band segmentation does seem to be based on an oscillation. And if you look at the stripes of Drosophila, there is a kind of feedback and remnants of oscillatory processes there. So even though the Drosophila segmentation process is very hard-wired with promoters of the same gene dedicated to individual stripes, the idea is that it didn’t originate that way. What it originated from was a Turing type reaction diffusion mechanism which then became hard-wired by subsequent genetic evolution. In this example, the short and long germ band insects employ what seemed to be entirely different mechanisms to make their segments, but if you look at it in physical terms, they have a common mechanistic origin. This idea is difficult to test experimentally, but Isaac Salazar-Ciudad, Ricard Solé and I showed its plausibility with computational modeling.
Katrina: What are the implications of these ideas surrounding physical mechanisms of development for our understanding of evolution?
Stuart: These ideas about more than genes being involved in morphogenesis certainly weren’t only my own. I was working informally with Stuart Kauffman and Brian Goodwin and there were other people who were in touch with physics and developmental biology and evolution. Since then these ideas have become well accepted in developmental biology; nobody believes now that it’s just a genetic program.
In contrast, the ideas about the role of physical mechanisms in evolution are not yet in the mainstream. You can accept the idea of physics participating in development and not necessarily draw conclusions from it that are at odds with Darwin’s gradualist theory. For natural selection to occur, you need variation within populations and it doesn’t matter how this variation generated; it can be through genetics, physics, or whatever. Then you have cycles of adaptation for fitness, and you have Darwin’s picture. You can add niche construction, for instance where organisms select the niche that maximizes their fitness, so that it’s not just the organism itself, but the organism in interaction with its environment. This doesn’t challenge Darwinism. But, if you look at how these physical mechanisms work to generate form, they’re very often abrupt changes, or nonlinearities. This is antithetical to Darwin’s gradualist theory and its modern versions.
You could say that even if you have an abrupt change, it still has to compete for resources and still has to establish itself by adaptive mechanisms. But if this abrupt change can lead to populating a completely different ecological niche – and a niche that may not have even existed before – then that’s very different from Darwinism because not only is the variation sudden, but the way of life is new. There’s a famous case of the bipedal goat. It was born without forelimbs, and its leg muscles seemed to rearrange in such a way that it could run around like a bipedal animal. If you have a cohort that has a sudden variation (like a group of bipedal goats, for example), they can explore completely new niches, and go places that other goats or other such animals never went before. This is not through gradual means and is unconnected to any cycles of incremental adaptation.
In retrospect you can say they’re adapted to their ecology, but the question is what is the mechanism of generating those adaptations? Is it a gradual fitness driven process? Or is it a kind of an opportunistic use of some novel component of the anatomy that might make it suitable to explore a completely new niche?
There’s another example in plant biology called transgressive hybridization where you have hybridization of two different genotypes. It leads to organisms that can be phenotypically extremely different from either of the parental strains. According to Ronald Fisher’s hypothesis, these hopeful monsters couldn’t possibly persist. But they do. They just go off and set up somewhere else and do perfectly well. They’re not competing within the pre-existing niche, they’re creating a new way of life that’s based on their new extreme morphology.
To me there’s nothing that can be more distinct from Darwin’s scenario for how you get biological complexity than getting complexity suddenly and not having to compete in an existing niche but going and creating a completely new one. Those two things put together, the suddenness of the variation and the unprecedentedness of the niche – is not the mechanism of evolution envisioned by Darwin or his successors.
The extended evolutionary synthesis (EES), as I understand it, accepts notions of developmental bias (non-random phenotypic variation) and heavily features niche construction, which is fully consistent with the view I have been describing. The EES also asserts that phenotypic plasticity can play an important role in evolution as a rapid, adaptive response mechanism that can guide evolution and precede genetic change. Where I differ is in the emphasis on adaptation, which I understand to be the notion of enhancement of relative fitness as a driving force of evolution and not just an after-the-fact description. In this respect, I don’t feel the EES has departed from the Darwinian paradigm as much as I believe is necessary.
Katrina: Let’s just clarify this. As I understand it, the EES has sympathy with the argument just described with reference to the bipedal goat, and would characterize this as plasticity first evolution. True, it does not argue that evolution occurs exclusively through plasticity first evolution, but it does maintain that this scenario may be far more widespread than generally conceived. Do you want to go further and argue that all evolution is plasticity first?
Stuart: Not at all. But I don’t think it’s just a matter of how prevalent plasticity first scenarios are. Plasticity itself is just a description of the capacity to exhibit a multiplicity of forms. Why does it have the specific outcomes it does in a given system? The real question for me is whether form follows function, or function follows form. The Darwinian idea is that the forms organisms assume result from improved reproductive success in the face of functional challenges. In contrast, if forms are inherent, e.g., if quadrupedality and bipedality are two intrinsic organizational modes of the same musculoskeletal system, neither of which required incremental selective trajectories to come into existence, then one or the other can emerge for reasons that that have nothing to do with any specific challenge. The Modern Synthesis, of course, is based on the idea that genetic mutations occur in this fashion, but it asserts that these are individually of small phenotypic effect.
If organisms can arise suddenly (through mutation or plasticity) that exhibit large phenotypic changes (Darwin called these “sports” and the mid-20th century geneticist Richard Goldschmidt, “hopeful monsters”) they can provide the basis for large-scale evolutionary change. Unlike the evolutionary ideas of Goldschmidt’s time that led to rejection of his notion, niche construction theory teaches us that these novel forms do not have to stand or fall in their originating ecological settings. Further, the physical understanding of morphogenesis we have been discussing here shows us that novel forms can be inherent to developmental systems and can thus be elicited in multiple individuals in a reproducible fashion.
Inherency (more on that later) is therefore not the same as plasticity but, the question you raise of the relative role of plasticity is also very important. You can’t necessarily judge this from the present-day world where you have organisms that are very highly evolved, very well established, and very well defending of their places in their niches. But in the world of half a billion years ago, who knows what kind of fluidity you had of habitat creation and morphological innovation, and so on. I would say that this mixture of sudden developmental variation that’s afforded by the physical mechanisms we have discussed, plus the possibility of creation of completely novel niches represents something very different from Darwin’s picture.
Katrina: Ok, I think I understand. The EES thus far has emphasized how novel phenotypes could arise through developmental systems responding flexibly to novel conditions, for instance through those exploratory processes emphasized by Kirschner and Gerhart. However, it hasn’t stressed how the action of physical forces inherently shape morphological features, sometimes in novel ways. Could you say some more about how you view plasticity?
Stuart: I think of plasticity as an inevitable consequence of the physical determination we’ve been talking about. Physics is a key factor in morphogenesis, and physics is subject to environmental parameters (things like temperature, pressure, and so on). So if you have forms that are generated by a collaboration of genetic and physical mechanisms, those forms will be plastic unless there is evolution to make them less plastic. I call this primitive plasticity.
Consider a segmentation mechanism that’s driven by these physical effects. You can show by experiments that such segmentation mechanisms are sensitive to temperature so that you get different numbers of segments at different temperatures. This is actually the case in centipedes, for example. You can also evolve genetic mechanisms that prevent the environmental perturbation of the number of segments. So you can build on this very plastic mechanism and make it more hard-wired so a species would only have a certain number of segments, and not a variable number of segments.
In studying a developmental process and you knock out what looks to be one or more important genes, you don’t always erase the morphology, but you make the morphology more variable. That can be an example of recovery of primitive plasticity, since you are removing some of the developmental reinforcers (canalizers) that have been acquired over time. On the other hand, there is evolved plasticity. Organisms can evolve away from rigid determination to become more plastic and more sensitive to the environment. When you see plasticity in an experiment, it’s sometimes hard to know which one you’re looking at. This distinction has been well discussed in the EES literature.
Katrina: Could you explain the concept of inherency and its importance in understanding evolution?
Stuart: Gerd Müller and I developed the notion of inherency and inherent forms early in our long collaboration. When we talk about inherency in development and evolution, we mean that certain aspects of morphology are latent in an embryo’s material identity (because embryonic tissues and organ primordia can behave as generic, or more properly, biogeneric, materials with predictable morphological outcomes), which therefore can appear in the course of evolution with very few steps. The predictability is key, because that is completely missing from the standard evolutionary picture.
When you first look at very complicated developmental processes and their morphological outcomes, it can be difficult to see what’s inherent about the forms. There’s a lot of elaboration in biology – you can have all sorts of ornate and elaborate appendages in insects, for example. You might acknowledge that there is physics involved in producing them, as we discussed, but you wouldn’t necessarily call those structures generic forms.
But it turns out that some developmental systems behave like non-living physical systems to so great an extent – the tissues of early animal embryos, for example – that their forms can be anticipated a priori. The cells of the tissue adhere to each other, and these sites of cell-cell attachment are also connected to the cytoskeleton, allowing coordinated motion. The cluster of cells then behaves like a liquid, and liquids have generic properties. We discussed tissue multilayering by liquid-liquid phase separation above. Another example arises when cells become polar, like you see in certain liquid crystals. Then you can get morphologies that are predictable from the liquid crystalline properties of the tissue.
The liquid crystalline phase is part-way between solid and liquid, where molecules are oriented in a predictable pattern like a solid, but the material is still able to flow like a liquid. Whereas drops of simple liquids are perfectly spherical (due to surface tension reducing the surface area to volume ratio), drops of a liquid crystal can become elongated due to the properties of the constituent molecules. A similar thing happens in animal embryos, where tissues can become elongated by a process called convergent extension. Cells become planar polarized and collectively align and intercalate. The tissue itself becomes elongated in a direction perpendicular to the intercalation of the cells, an inherent outcome of the liquid crystalline state.
Still another case is when polarization involves differences in adhesive properties across the surfaces of cells – apicobasal polarity. In the multicellular context this leads to hollow structures where the less adhesive parts will be interior. This kind of self-organization is familiar from the behavior, at a smaller scale, of phospholipids in the formation of membrane vesicles. D’Arcy Thompson, in the early 20th century, and William Bateson before him were captivated by the idea that living tissues generate predicable forms because physical laws that apply to nonliving materials also pertain to them. Much of my work has been an attempt to meld those earlier ideas with modern cellular and molecular biology.
The concept that cells and their genes produce forms during development and evolution by mobilizing physical forces and effects has proved extremely fertile. For example, my colleagues Ramray Bhat and Tilmann Glimm and I have used a combination of experimentation, comparative phylogenomics and mathematical modeling to frame a plausible scenario for the rewiring of patterning mechanisms during the fin-to-limb transition in vertebrates.
By understanding the physical bases of morphogenesis, you can anticipate what kinds of forms will appear. If the developing organisms are acting as a generic material (like a liquid, like a liquid crystal, or in the case of plants, like a deformable solid), you could say that the forms, even though they don’t all appear in evolution at the same time, are inherent to the materials. A good analogy for this is the fundamental particles that make up the chemical elements – the electrons, the neutrons, and the protons. All the chemical elements didn’t appear at one time in the history of the universe; they appeared sequentially, based on each other through collisions and so on. But you could say, based on quantum mechanics, that the 110 or so elements that we see are generic to those particles in interaction with one another. In other words, you can’t get anything in-between and you can’t get much beyond.
If you look at the biological world in this way, what could be more anti-Darwinian than the idea that the forms we see in multicellular organisms are actually inherent to cellular clusters? In this view, cycles of selection will just select among things that are latently there anyway, and that emerge spontaneously, or nearly so. Recognition of this kind of inherency is missing from the statements that I read of the extended synthesis. In my view, when you bring that in, the synthesis is no longer just extended; it’s something new.
Katrina: So your view would push beyond extending the synthesis, to creating a new one?
Stuart: Yes. That new one doesn’t deny the insights of Darwin but it maybe puts those mechanisms a little bit on the margins; maybe they’re not always the important mechanisms. I think the Darwinian mechanisms can be sustained for micro-evolutionary changes. But for macro-evolution – going from unicellular to multicellular, or going from multicellular to hollow, elongated structures with segments and appendages, and the corresponding motifs in multicellular plants – I don’t think you really need Darwinian mechanisms.
Katrina: That’s very interesting. Thank you.