The variational ratchet: Intuiting the variational approach to niche construction
by Axel Constant & Karl J Friston
17 May 2018
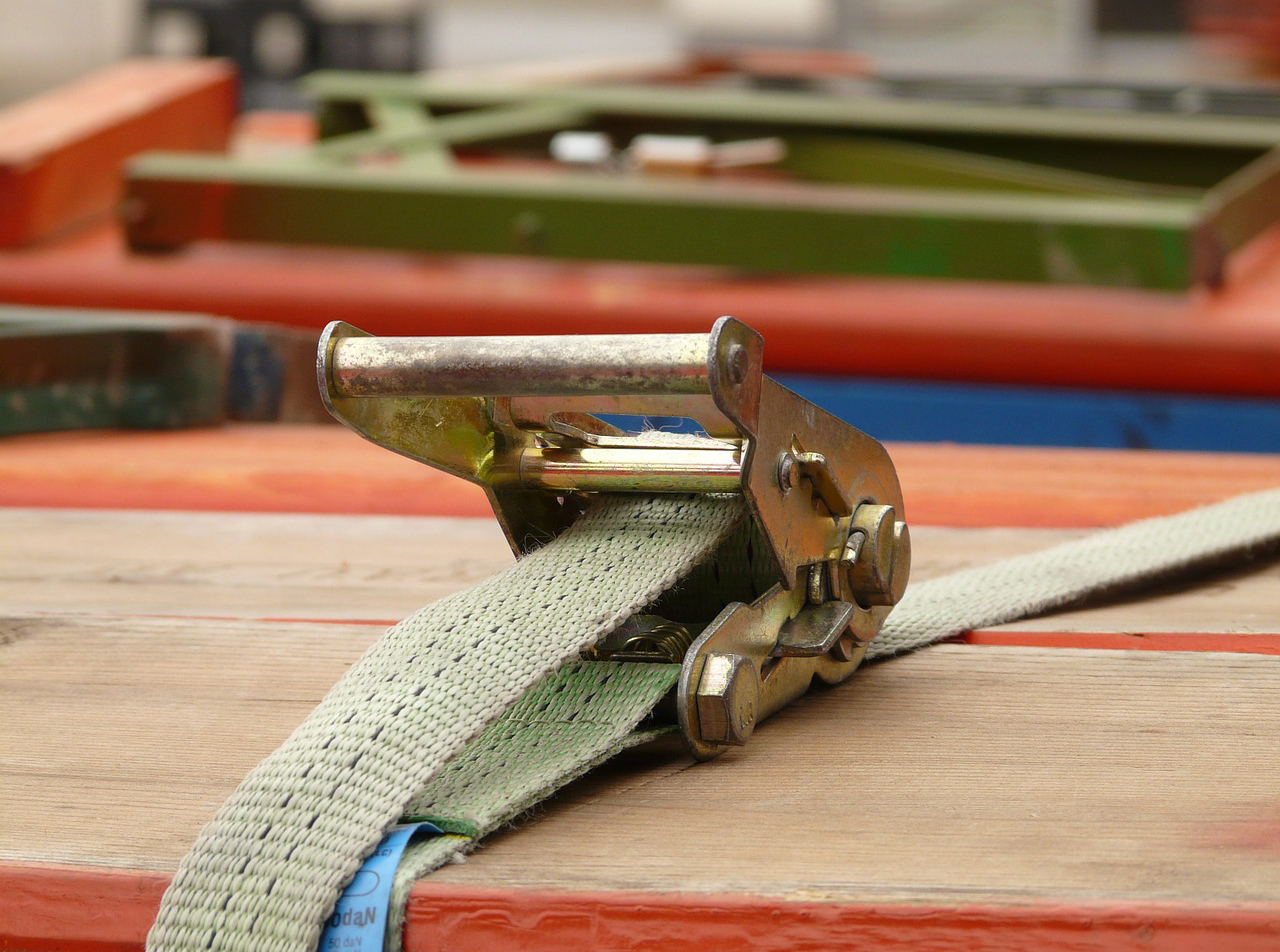
The principle of evolution by natural selection provides a solid conceptual tool to understand adaptive design. It operates like a ratchet, to retain and build upon functional variation. Pull down, ‘click’! Variation. Hold tight, lock it! Retention and differential fitness. Push up! Inheritance, and ratchet your way up towards peaks in the adaptive landscape. As this process is repeated over phylogenetic time, the organism-environment complementarity will tend to get tighter and tighter, often leading to enhancements in the properties of adaptations.
But this ‘Darwinian ratchet’ is not the only tool that nature uses to create adaptive design. Often implicitly, organisms themselves crank the ratchet, decide where to lock it in, and so shape species’ trajectories throughout the adaptive landscape. In effect, through their normal bioregulatory activity (e.g., metabolism, dietary choices, waste production, construction of nests/webs/burrows, etc), organisms modify their environment, and in so doing, influence their own evolutionary trajectory, and that of other species. This process is studied by niche construction theory.
For instance, organisms can produce niche construction outcomes like beaver dams that can directly increase the fitness of the genes underlying dam construction (known as extended phenotypes); produce ecological inheritance, like beaver lakes, lodges and modified communities, which can support the reproduction of the adaptive life cycle for beaver kits; and engender ecological cascades, for instance, caused by the construction of beaver dams, which can force novel evolutionary responses by perturbing the environment.
The variational approach to niche construction (VANC) reads niche construction theory under the lens of the variational free energy principle, as applied to life sciences. With this principle, it is fairly easy to show that mathematically, if an organism can be distinguished from its environment (that is, it does not dissolve, dissipate or decay into its environment when facing environmental perturbations), then it must behave as if it is trying to maximize its statistical attunement with its environment. This view finds roots in the works of people like Hermann von Helmholtz and Ross Ashby, for whom modeling and inference were necessary attributes of living, self-organized systems enduring over time.
Put another way, organisms must increase the complementarity of the probability distribution embodied by their phenotypic states (e.g., body temperature, or brain states), and the probability distribution of states defining their environment (e.g., ambient temperature gradients), causing their sensations (e.g., sensory inputs conveyed through sensory organs). For instance, when animals are cold, they might move towards a heat source, or perhaps find or build a shelter, to gather sensation consistent with their expectations, thereby maintaining themselves within their most likely set of phenotypic states.
Organisms secure their complementarity through action (and sensory perception) and environmental modifications, or ‘active inference’. Broadly construed, active inference involves (i) the update of embodied expectations based on perceived sensations; (ii) the selection and realization of those newly updated expectations via physical action, and (iii) correlatively, the implicit (or explicit) production of environmental modifications consistent with those expectations. These modifications can be manifest in the short term (e.g., cutting down a tree) – or long term (e.g., building a dam).
A curious twist here is that a similar argument can be applied to the environment. Because of the nature of active inference, over time, regularities in the organism’s behavior become embodied in the environment, the same way that the organism ends up embodying regularities of the environment. For instance, in navigating their environment, earthworms modify soil constitution in a way that suits their physiology and behavioral patterns. They build tunnels, which in turn, take the shape and properties of those typical, or expected physiological and behavioural traits that led to the production of the tunnels in first place. In short, earthworms and tunnels, beavers and dams, all are in the game of ‘reciprocal modelling’. We can think of this in terms of generalized synchrony; e.g., two swinging pendulums attached to a beam that will inevitably synchronize over time. Put simply, the organism models its environment which, in turn, models it back, and we end up with a world that reflects the organism’s expectations. Of course, in reality the appearance of counter-modeling by the environment is a consequence of the organism’s activity. The environment cannot inherently evolve an inferential capability, but (as a consequence of the organism’s activities) the environment will nonetheless change over time to take on a form that captures the expectations that the organism has about its environment and/or regularities in the organism’s behaviour. VANC describes the forms that the environment takes via niche construction as expectations (or Bayesian estimates) about the organism’s activity, in the same way that it describes the organism’s expectations about its environment in Bayesian terms.
The consequences of this are, on the one hand, that at the ontogenetic time scale, organisms often implicitly identify relevant components of their environment (e.g., the residues of other organisms’ foraging activity), the learning from which will enable conspecifics to become accurate or adapted statistical models of their environment (e.g., becoming good palm nut crackers). On the other hand, at the intergenerational time scale, organisms will pass on these relevant components through ecological inheritance, which will train future generations to acquire and embody an accurate model of their environment or recapitulate the adaptive life cycle (e.g., by the implicit flagging of proper material for nutcracking based on the accumulation of nutshells and oily residues at certain sites, and absence of these cues at other locations not conducive to nutcracking).
On this view, the life-cycle is just an orbit through some immensely high dimensional state-space that ensures that the phenotype (or species) does not dissipate – and can therefore be distinguished from its environment, in virtue of returning to characteristic states. In short, the very existence of cycles within cycles (i.e., orbits within orbits) just is active inference – that looks exactly like self-organization (in dynamical systems) and self-assembly (in molecular biology), depending upon the scale of interest.
The fundamental intuition behind the VANC is that, under active inference, niche construction can be seen as another ‘evolutionary ratchet’ that can be used to explain adaptation. The expectation updates, action selection, and environmental modifications, all conspire to maximize the tightness of organism-environment ‘statistical’ complementarity, as scored by variational free energy. The activities of organisms modify environmental states in such a way as to increase organism-environment complementarity, often in ways that in the process make it easier for future organisms, particularly descendants, to model the environment.
An outstanding question remains: can we model the effect of ecological cascades on organism-niche complementarity with the VANC, in the same way that we model organism-derived environmental modification during ontogeny? It seems so! We leave you with some exciting results from our most recent VANC simulation. Our results were obtained by simulating a target reaching task in the context of environmental navigation (in a maze made of an 8×8 black and white grid). Each move entailed an implicit modification of the sensory features of the environment consistent with the organism’s expectations (e.g., turning a black location into a whiter location for organisms that preferred white locations), and led to changes in the expectations of the organism concerning the perceptual features of the maze. Each location was associated with a value that captured ecological inheritance (e.g., energy/matter reported by the color of the location) and semantic information (e.g., ‘know how’, such as how to crack open palm nuts, or in our simulation, the extent to which a location attracts the organism towards paths leading to the target location). Each new generation inherited the environment of the previous generation, and the complementarity between the distribution of states of the environment and the organism was scored by variational free energy.
Our results suggest that organismically induced cascades of ecological inheritance negatively impact the complementarity of developing conspecifics when that inheritance is uninformative (i.e., semantically poor), but yields the highest level of complementarity when the inheritance is informative (i.e., semantically rich). Moreover, over multiple generations, cascades of ecological inheritance increasingly drove the exploration of the maze, and forced a variety of complementarity solutions (e.g., distinct configurations of expectations and patterns of environmental modifications) to the problem posed by semantically poor ecological inheritance.
For more detail, read the paper here:
Constant A, Ramstead MJD, Veissière SPL, Campbell JO, Friston KJ. 2018. A variational approach to niche construction. J R Soc Interface 15:20170685.
[pdf]
Or email the authors:
Axel Constant
Karl J Friston