Epigenetic inheritance in evolution: an interview with Eva Jablonka
by Eva Jablonka & Katrina J Falkenberg
11 July 2017
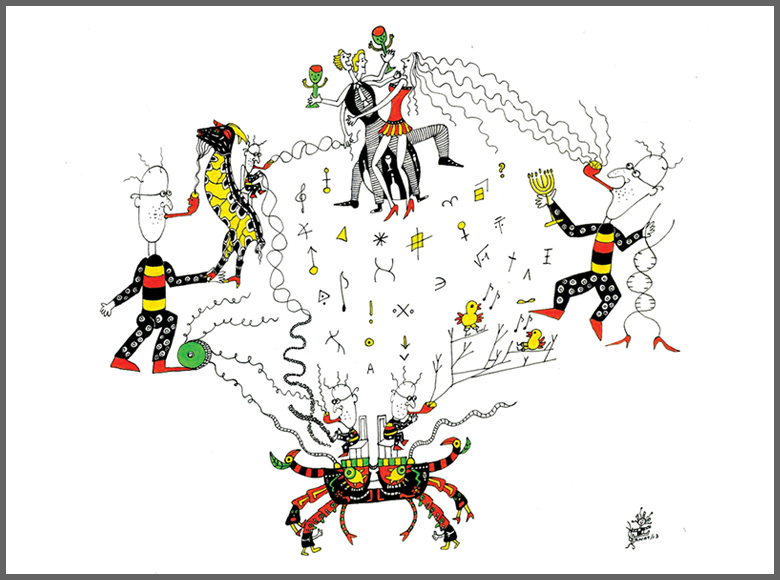
Eva Jablonka is a professor at The Cohn Institute for the History and Philosophy of Science and Ideas at Tel Aviv University, Israel. With Marion Lamb, she has been the most prominent longstanding champion of the importance of epigenetic inheritance in evolution and has published many papers and books on the topic. Katrina Falkenberg is the science communication and outreach officer for the EES research program and she interviewed Eva about transgenerational epigenetic inheritance and its importance in adaptive evolution.
Katrina: How would you respond to the following criticisms of the importance of epigenetic inheritance in evolution?
Common criticism #1 – Epigenetic variation is under genetic control.
Eva: Well, I think it’s a funny kind of criticism because cultural variation is also under genetic control in the sense that it depends on the presence of the genetic system. In this sense, everything in organisms on this planet is under genetic (nucleic acid) control. That doesn’t mean that the system cannot be autonomous of genetic variation. It depends on genetics, but it doesn’t have to depend on genetic variation. This is something that people mix up, I think. Cultural evolution is a real process and people agree that in this case there is an independent axis for cultural evolution, based on cultural rather than genetic variation. The same is true, at least in principle, for epigenetic evolution based on the inheritance of epigenetic variations.
Katrina: Common criticism #2 – Epigenetic variation is too unstable (reversion rate too high) to allow variants to spread through a population and go to fixation.
Eva: There are several answers to this question, and they are not mutually exclusive. We must remember that not all evolutionary change involves the fixation of variants. The first and simple answer to this questionable claim is that even if the inheritance is not very stable it still leads to population dynamics that are very different from those you would have if you didn’t have epigenetic inheritance. This has been modeled1-5 and there is no question about this. You cannot understand evolutionary change, even changes in the genetic constitutions of populations, without incorporating the dynamics introduced by epigenetic inheritance. Even if the transmission of epigenetic variations is only short term, let’s say only four generations, you will still get completely different dynamics from those you would get if you did not consider it. Nobody who knows anything about modeling will challenge this.
The second answer is that we still know very little about the stability of epigenetic inheritance and what we do know suggests that it is very variable. Sometimes it is unstable, sometimes it is stable. In the flowering plant, Arabidopsis thaliana, the rate of change of methylation at single CpG sites (cytosine-phosphate-guanine; a cytosine nucleotide followed by a guanine nucleotide) is something along the lines of 4×10-4 per generation6. This is much, much higher of course than the rate of mutation in coding genes, 5 orders of magnitude in fact7, but it’s low enough to be selected and lead to cumulative evolution6. The relatively high rate of epimutation is similar to that of short tandem repeats (STRs) in DNA8, which are acknowledged to play an evolutionary role. We don’t know very much about what modulates the stability of transmission but I think it’s context and history dependent, and if you accumulate epigenetic variation through many generations of repeated induction of epigenetic variation, it may can become increasingly stable. This is an interesting and important research question.
A third point is this: selection and induction usually go together. Often induction and selection are features of the same environment, so the selecting environment is the same as the inducing environment. If you change the inducing/selecting environmental conditions you are selecting those who can withstand the change but you’re also inducing all kinds of epigenetic changes in gene expression and developmental mechanisms in the relevant genes. The gene expression patterns that give you some ability to withstand this environment are selected for during development, and some of these induced developmental variations can be inherited between generations and will be selected.
Epigenetic inheritance will not be effective if the environment changes all the time. What can you select for in such conditions? Because the selective conditions will be changing all the time, you will not get fixation of any genetic or epigenetic variant. Additionally, if the transmissibility of epigenetic variants is not very high, you will always get epigenetic polymorphism. This is a kind of bet-hedging strategy, which is adaptive in fluctuating conditions. On the other hand, if the environment is stably inducing and selecting for many generations, it is very likely that in due course, you will have genetic variation that will support the existing selected but less stable epigenetic variation. Then you will have genetic assimilation of the character and partial phenotypic assimilation. So genes, as Mary Jane West-Eberhard would put it, will follow the epigenetic adaptations in such cases.
Katrina: Common criticism #3 – There is little evidence that epigenetic variants have adaptive benefit, in fact they are usually neutral or deleterious.
Eva: This is nonsense, first because there are already many examples for adaptive or potentially adaptive epigenetic variations (for example, in flowering time and root length in plants) and second, because of discovery bias: when we discover a new thing it is often because we encounter problems. It was exactly the same in the early period of genetics. The first mutations that were discovered all had deleterious effects. That is why, in the beginning of the 20th century, some people thought that Mendelian genetics was not important. They argued that since all these mutations they saw have deleterious effects they cannot be the basis of adaptive evolution. It’s a nice, interesting historical analogy to some of today’s claims about epigenetic inheritance. But I want to stress – we have enough examples to suggest that epigenetic variation can lead to adaptations that are based on epigenetic variations. A good example is resistance to viruses in the nematode Caenorhabditis elegans9, through a transgenerational small RNA-based cellular immunity. Another is resistance to activities of transposable elements through DNA methylation in, for example, the fungus, Neurospora10. In these cases, adaptive heritable change is brought about through epigenetic mechanisms and the transmission of epigenetic variants. In plants, you have, as I mentioned earlier, epigenetic differences that affect root length and flowering time11. Surely such traits can have adaptive advantages in some environments.
Another thing to think about is adaptive improvisation12, or exploratory behaviour, coupled with stabilization of adaptive solutions. Environments change in ways that are to some extent unpredictable and organisms have to respond to these new, unforeseen, challenges. They engage in exploratory behavior at the level of basic metabolism in cells, at the level of larger scale physiological systems, at the behavioral level, etc. Epigenetic exploration can rewire gene regulatory pathways in novel environments. There is a nice study on the yeast, Saccharomyces cerevisiae, by Braun and colleagues investigating this13. They engineered yeast cells so they couldn’t synthesize the amino acid histidine, which they need for them to survive and to divide. In the absence of histidine synthesis, which was supressed in these cells, there was a lot of trial and error within the cells, a lot of continuous wiring and rewiring of metabolic circuits. Finally, changes in gene regulation that allowed the cells to divide were “discovered” by the cells, and were stabilized and inherited for hundreds of generations. This is inheritance through epigenetic inheritance systems but we don’t, as yet, fully understand which mechanisms are involved.
In other cases, we know a little bit more about the mechanisms. We know that in the case of the fruit fly, Drosophila melanogaster, where the same kind of experiments were done, that epigenetic inheritance at the level of the chromatin binding proteins is involved14,15. What this means is that epigenetic variation within a cell, can lead to a positively reinforced response (which is developmentally selected), and then this response can be inherited by a new generation of cells or organisms (even if they are multicellular organisms). The story is more complicated because it also involves selection of the flies’ microbiome.
To answer another part of your question: yes, some may claim that epigenetic variation is often/usually selectively neutral. Indeed, variation in DNA methylation at single CpG loci can often be selectively neutral, just like genetic variation in short tandem repeats (STRs), but one must remember that some changes in the highly mutable STRs do have phenotypic, selectable consequences, and so can changes in single CpGs that vary at the same rate as STRs. However, there are differentially methylated regions (DMRs), that are clusters of CpG-containing repeats, in regulatory regions. Altering the methylated state of several CpGs may be necessary for altering the regulatory effect of the DMR, and in these cases the rate of change will be much lower.
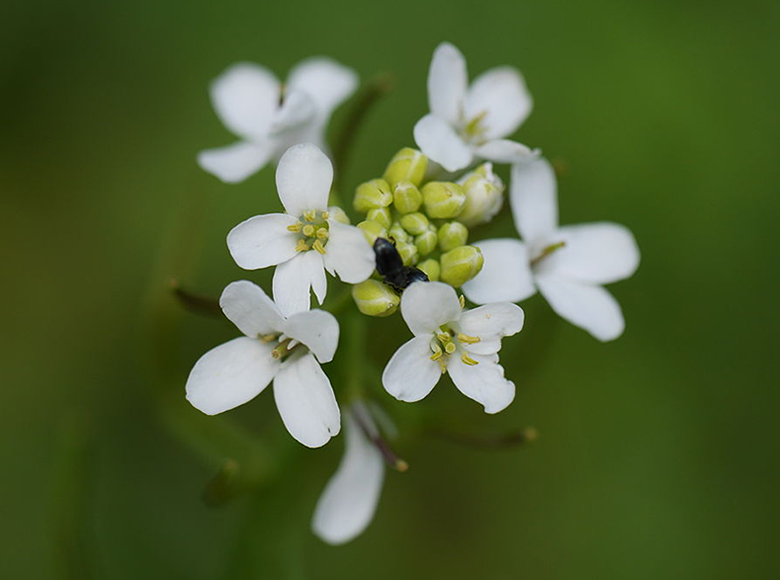
Arabidopsis thaliana (image credit: Dawid Skalec, CC BY-SA 4.0)
Katrina: Is there a simple way to describe the most important classes of epigenetic inheritance?
Eva: Marion Lamb and I tried to classify epigenetic inheritance mechanisms into four major groups16,17 but they often work together:
• small RNA-mediated system
• chromatin marking
• self-sustaining loops
• three dimensional self-templating
Different organisms use different mechanisms to different extents. In Drosophila, for example, it seems like there is very little DNA methylation. It uses histone modifications, it uses the small-RNA system and of course like every organism we are aware of, it uses self-sustaining loops. We are beginning to know a little bit more about prions (three-dimensional self-templating), for example there was a recent paper about prions in bacteria18. These mechanisms are more or less universal but of course their use was honed by selection in different groups and there are good reasons for that. I will not go into that now but Aviv Regev, Marion Lamb and I wrote a paper in 199819 trying to figure out why some groups have DNA methylation and some groups don’t, what could be the reasons for that, and what we could infer about the role of these systems. I think that this is a very interesting area of research.
Katrina: What fraction of genes are affected by heritable epigenetic mechanisms? Are these important functional genes or simply transposons or other repetitive elements?
Eva: The best work has been done in plants. In Arabidopsis thaliana, we have more than 100,000 CpG sites that are changed due to DNA methylation. Some of them are transposons, some of them are not, and the more stable heredity is actually found among euchromatic genes that are not transposable elements6,20-22. And that’s just talking about one type of epigenetic variation, the DNA methylation system. DNA methylation depends on the structure of the DNA; whether you can put methylation down depends on whether you have for example, CpG sites (although there are also other forms of methylation for example, such as CpNpG sites that can be methylated). There are other modifications of DNA as well – you know, we’re only scratching the tip of the iceberg – and we don’t know how these are distributed across the genome. Probably most of the sites in the genome can be, under some conditions, silenced with small RNAs. There are also histone modifications which are all over the place and how stably these are inherited we don’t know in most cases. All in all it seems that most DNA sequences (particularly regulatory sequences) can assume variant epigenetic states, and that some of these can be inherited under some conditions. There is a lot of research that needs to be done.
Katrina: For mechanisms of epigenetic inheritance to be important, must they have gene-like properties, or can they still play an important role in evolution if they are unstable?
Eva: No, they do not need to have gene-like properties, although some do. DNA methylation is the closest to having gene-like properties because you have a kind of dedicated copying, replication-like, mechanism. In other cases, you have reconstruction, not blind copying or semi-blind copying mechanisms. Marion and I wrote about it in the dark ages, in 199516, and nobody took any notice for a long time. Now it’s clear that some epigenetic mechanisms are not replication mechanisms; they are reconstruction mechanisms.
One has to remember that very often the different mechanisms of transmission of epigenetic variations interact; there is a kind of synergy among them. So when you are looking at stability of just DNA methylation, you may find that it’s not very stable and when you’re looking at the phenotype, you can see that it is stable (in heredity). And you ask yourself, “why is the phenotype stable when the methylation of the relevant gene isn’t’?” And you will find out that it’s because it’s not just methylation. We know that the methylation system and the small RNA system and the histone modification system are very, very closely intertwined, so DNA methylation can lead to the formation of RNAs that are transmitted, that reconstruct the methylation pattern, that leads to synthesis of RNAs and so on, so at some points in development you will see that a particular epigenetic factor has disappeared. We don’t know enough about the epigenetic events that occur in the germ line, and this is another important area of research. What we do know is that it’s a really nice, rich complicated story. Sometimes a particular type of variation, say in DNA methylation, is sufficient for the phenotypic change and its heritability, sometimes it is not sufficient but necessary, more often it is facilitatory rather than necessary and sufficient. Generally we have to isolate a system in order to be able analyse it, but if we’re asking a question at the phenotypic level, we have to reconstruct the whole system.
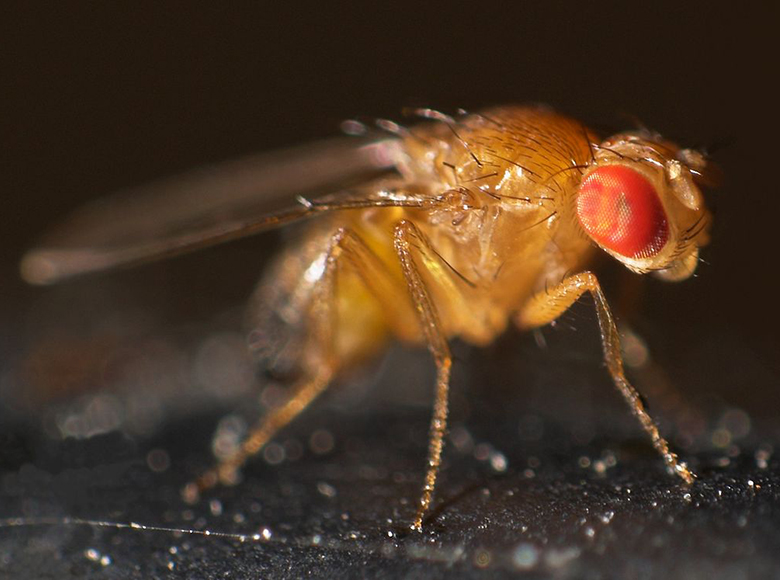
Drosophila melanogaster (image credit: Mr.checker, CC-BY-SA-3.0)
Katrina: Some people would argue that there is nothing special about DNA methylation; it’s basically just a fifth base. Do you agree?
Eva: That’s a very interesting point, it surely makes life easier for some of the critics. You could treat it as a fifth base in the sense that you can sequence the methylome, treating methylated cytosine as a fifth base, consider the rates of forward and backward methylation and so on. But it’s a peculiar fifth base, isn’t it? It doesn’t change coding properties and it is affecting gene expression, and in this sense it is rather different from the other bases. You can call it whatever you like. If you want to call it the fifth base, fine, but you would have to admit that it has a role in both transmission and expression: it can be both copied by methyltransferases and affect gene expression, all at the same time. Since variations in DNA methylation pattern can be sensitive to the environmental conditions and may lead to heritable variations in gene expression, there is a potential for soft inheritance here, for Lamarckian inheritance.
Katrina: Now for a sociology question: why is there so much resistance (from geneticists) to the inheritance of acquired characteristics? Is this the ghost of Lysenko?
Eva: Well it depends. I think a lot of people are very invested in a way of thinking that they’ve been socialized in. They see things in this way and it’s very difficult to do the kind of gestalt switch you need to do in order to see things differently. So I think that’s the first answer; it’s really difficult to change one’s point of view. The other thing, I think, especially in the United States, is that scientists are afraid if you criticize the classical neo-Darwinism or Modern Synthesis, you will encourage the creationists. They are sort of primed to ‘defend the citadel’ – I think that’s another reason.
I think that the Lysenko issue is much less important now. In fact most young people have never heard about him. Lysenko was a charlatan and he did a lot of damage to people and science; he was an awful man living in awful times. He misused ideas, he cheated, he was a terrible scientist. But there was some real data at the Lysenko time in the USSR, produced by honest and good scientists who were very interested in stress and the effect of stress on heredity, which suggested that changes in the environment can have heritable effects. Some of this research was very good, some of it was not. Because it occurred during the Lysenko period it was ignored in the West. It was assumed fraudulent by association.
It is sobering to remember that the same kind of guilt by association can be applied to Mendelian genetics because of the misuse of Mendelism by eugenics. Early eugenicists told us that every trait – cognitive, emotional or whatever (for example, if you’re a good church goer or not), is dependent on a single locus controlled by recessive or dominant alleles, and its inheritance follows Mendelian laws. Horrible things happened in the name of eugenics. The political fact that eugenicists misused Mendelism to serve their political ends doesn’t discredit the basic facts of Mendelism. Similarly, Lysenkoism should not discredit epigenetics and epigenetic inheritance. There was a cold war between the USSR and the West, and there was also cold war in biology. There are still echoes of that today. I do hope we can go beyond this.
Katrina: Final question: can you comment on the recent paper from Charlesworth et al23, which claims that “allele frequency change caused by natural selection is the only credible process underlying the evolution of adaptive organismal traits”?
Eva: There are a number of problems with this paper, many of which we have already discussed. There are three final points I’d like to make regarding the arguments in this paper:
- Charlesworth et al bring up the role of selection (section 4c). The authors claim a causal role for epigenetic inheritance in adaptive evolution requires that “natural selection is not involved in the spread of such [epigenetic] variants through populations”. This is not true. Selection is always important for adaptive evolution, whether it be acting on genetic or epigenetic variants. The authors did not discuss different forms of selection, for example, developmental selection followed by natural selection within populations is never addressed. Epigenetic inheritance facilitates and can bias adaptive evolution, especially when developmental selection is included.
- The evolutionary origin of epigenetic inheritance mechanisms is not necessarily relevant to current function. These mechanisms probably evolved for genome defense against ‘genomic parasites’ and regulation of expression and transmission. The fact that epigenetic inheritance may be an evolved strategy does not detract from its value as a semi-independent axis of evolution; just as the capacity for cultural evolution is evolved but this does not detract from the efficacy of cultural evolution and cultural adaptations.
- Charlesworth et al note that “a large body of genetic experiments has established the ineffectiveness of selection on homozygous lines, which lack genetic variation but still show phenotypic variation.” This is hardly surprising because such lines are generated through either self-fertilization or brother-sister mating, and all chromosomal variability – both genetic and epigenetic – is greatly reduced. Heritable selectable variations are gradually built up when a population is established. The phenotypic variation in homozygous lines is initially the result of the de-canalization of development, which typically leads to stochastic and transient phenotypic variations (although occasionally it may lead to heritable epigenetic variations: there is, not uncommonly, some residual selectable variation in inbred lines). The question is: when selectable variation is eventually generated, is it genetic or also epigenetic? The assumption was that it is all variation in DNA but the possibility of (additional) epigenetic variations was not tested.
Eva Jablonka discusses some of these topics (and others) in the following paper:
The evolutionary implications of epigenetic inheritance
Jablonka E 2017. Interface Focus 7 20160135; DOI: 10.1098/rsfs.2016.0135
1. Day T & Bonduriansky R. 2011. Am Nat 178:E18–E36. 2. Geoghegan JL & Spencer HG. 2012. Theor Popul Biol 81:232–242. 3. Geoghegan JL & Spencer HG. 2013. Theor Popul Biol 83:136–144. 4. Geoghegan JL & Spencer HG. 2013. Theor Popul Biol 88:1–8. 5. Geoghegan JL & Spencer HG. 2013. Theor Popul Biol 88:9–19. 6. van der Graaf A et al. 2015. Proc Natl Acad Sci 112:6676-6681. 7. Ossowski S et al. 2010. Science 327:92–94. 8. Fan H & Chu JY. 2007. Geno Prot Bioinfo 5: 7–14. 9. Rechavi O, Minevich G, Hobert O. 2011. Cell 147:1248-1256. 10. Aramayo R & Selker EU. 2013. Cold Spring Harb Perspect Biol 5:a017921. 11. Cortijo S et al. 2014. Science 343:1145-1148. 12. Soen Y, Knafo M, Elgart M. 2015. Biol Direct 10:68. 13. Braun E & David L. 2011. MIT Press pp 181-191. 14. Stern S, Fridmann-Sirkis Y, Braun E, Soen Y. 2012. Cell Rep 5:528-542. 15. Fridmann-Sirkis Y et al. 2014. Front Genet 5:168. 16. Jablonka E & Lamb MJ. 1995. Oxford UP. 17. Jablonka E & Lamb MJ. 2005. MIT Press. 18. Yuan AH & Hochschild A. 2017. Science 355:198-201. 19. Regev A, Lamb MJ, Jablonka E. 1998. Mol Biol Evol 15:880-891. 20. Teixeira FK et al. 2009. Science 323:1600-1604. 21. Johannes F et al. 2009. PLoS Genet 5:e1000530. 22. Schmitz RJ et al. 2011. Science 334:369–373. 23. Charlesworth D, Barton NH, Charlesworth B. 2017. Proc R Soc B 284: 20162864.